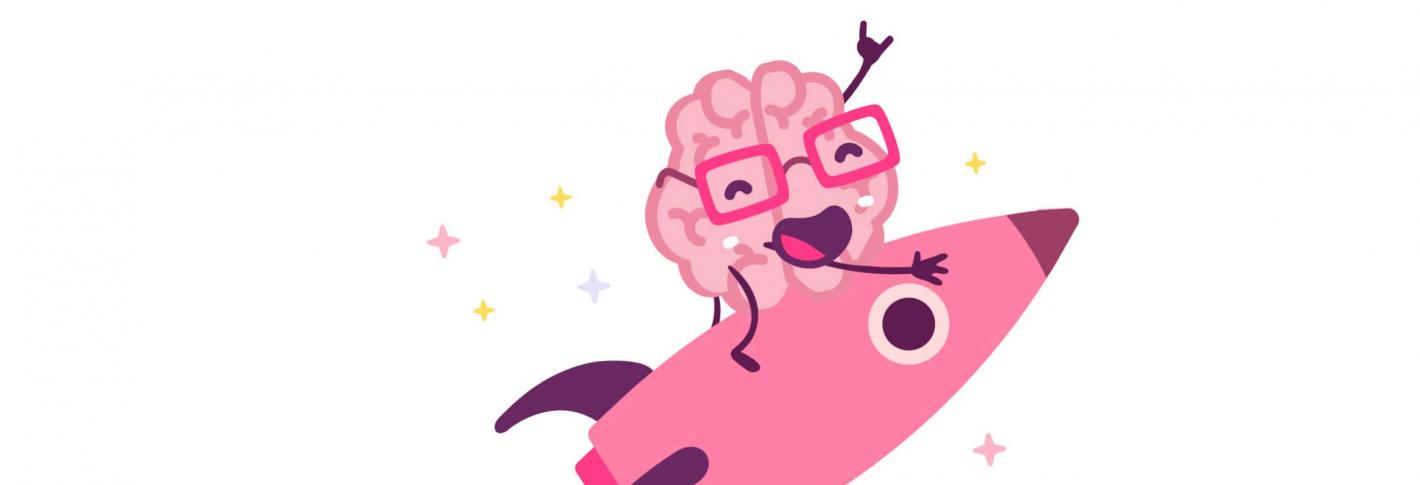
Graduate student Hunter Iaccarino came to the lab of Institute Director and Picower Professor Li-Huei Tsai eager to try an idea that circa 2014 seemed somewhere between provocative and preposterous. Aware that 40Hz frequency brain waves were reduced in Alzheimer’s disease patients and mouse models, and knowing that the Alzheimer’s-focused Tsai lab had shown how to increase the power of exactly those 40Hz ”gamma” rhythms, Iaccarino figured Tsai would take a chance on testing whether boosting the rhythms would affect the course of the disease.
Never mind that the conventional wisdom assumed that rhythms were nothing more than indications of brain activity, not anything worth attempting to manipulate in hopes of profoundly affecting an incurable and devastating brain disease. From that viewpoint, altering rhythms to treat disease should be no more consequential than trying to fix a car’s motor by fiddling with the “check engine” light..
So what could Tsai and Iaccarino do to get this unprecedented and untested idea off the ground? They couldn’t just apply for a traditional government grant. Those require solid preliminary data. Trying this untried idea would instead require private, philanthropic support. In fact, many of the boldest, riskiest ideas in the Picower Institute, and in science at large, get their start because of private gifts and awards, either from foundations or individual donors who know that true innovation requires investment, sometimes in people more than projects, and that investment carries risk.
And so Tsai and Iaccarino started up the very first experiments by drawing on funds provided to the Picower Institute by its naming benefactor, the JPB Foundation of Barbara Picower. Once they began to see, and confirm, the earliest results—that boosting 40Hz rhythm power substantially reduced protein plaques that are hallmarks of Alzheimer’s pathology—with the help of former MIT Chairman Robert Millard and his wife Bethany, they showed them to donors including Jeff and Nancy Halis who helped propel the early research forward, culminating in a landmark paper in 2016 and more papers since. More seed funding from many donors including the Eleanor Schwartz Charitable Foundation, the Degroof-VM Foundation, and the Halis, DiSabato and Siegelman families launched human clinical studies that have now shown significant therapeutic benefits. Moreover, it has proved that manipulating rhythms can have direct effects on cellular and molecular processes of Alzheimer’s disease.
“Our research on gamma rhythms was completely launched with philanthropic giving,” Tsai said. “Long before new research typically can get grants from the standard programs at the government agencies, private support is essential.”
Maybe Marmosets
What’s true for studying neurodegenerative disease is also true for studies of autism spectrum disorders. A few years ago Newton Professor Mriganka Sur and colleagues in MIT’s Brain and Cognitive Sciences department realized that to better understand the fundamental neuroscience of autism, they needed an animal model more cognitively and socially sophisticated than mice, but less logistically challenging than rhesus macaque monkeys. The answer, they realized, might be marmosets. About the size of large squirrels, marmosets are highly social primates that are more easily housed than macaques, reproduce much more frequently, and produce larger litters (an important need when breeding genetic research lines).
“When it comes to primates, they are probably the best possible testbed for understanding autism,” Sur said.
Maybe, but no one had shown that marmosets exhibit behaviors and underlying cellular and circuit mechanisms with meaningful relevance to autism. To become the first, Sur teamed up with fellow MIT Professors Ann Graybiel, Bob Desimone, and Alan Jasanoff. How could they get started attempting to develop a brand new animal model for autism? They brought together private support from the Simons Center for the Social Brain (an MIT program Sur directs with funding from the Simons Foundation Autism Research Initiative), the Stanley Center at the Broad Institute, and the Hock E. Tan and Lisa Yang Center for Autism Research at MIT’s McGovern Institute for Brain Research.
Each lab is now testing different aspects of marmoset neurophysiology and behavior. Sur’s lab, for instance, is finding that marmosets are very good at playing a game that tests their ability to form expectations and make predictions. After seeing a picture of a fellow marmoset on a screen, the animal can get a reward if it taps the screen as soon as possible after the image disappears. Success stems from learning to predict when the image will disappear (a probability set by the researcher). All the while the scientists measure brain activity via electrodes on the marmoset’s head to see which brain regions are involved in its mental calculations. The next step will be to test what happens when marmosets engineered with autism-associated mutations try the game. Will they show a similar difference as people with autism? Will it be because of similar activity changes in the same brain regions?
In parallel, Graybiel is looking at how activity in a region called the striatum may contribute to abnormally repetitive behaviors, Desimone is looking at how marmosets perceive faces, and Jasanoff is tracking brain activity evoked by social cues.
“If this takes off it will lead to a new model for neuroscience. But it could all fall flat.”
All these experiments are unprecedented in marmosets but if they are successful, then the team will have introduced a powerful new autism animal model. Sur envisions MIT becoming a global resource for marmoset-based studies of autism.
“If this takes off it will lead to a new model for neuroscience. But it could all fall flat,” Sur said. “Private foundations or internal sources of funds are essential for carrying this work forward.”
Making a Mark on Fragile X
Today Picower Professor Mark Bear is a leading authority on the neurobiology of fragile X syndrome, the most common inherited form of autism, but back when he made a seminal discovery about the disorder, he had no familiarity with it at all.
Bear’s lab had been working to understand the molecular means by which circuit connections between neurons would weaken, a process called “long-term depression,” or LTD. He and postdoc Kim Huber had found that activating a receptor called mGluR5 triggered LTD, and that it could be constrained by a protein called FMRP. Aware that silencing of the FMRP gene causes Fragile X, it dawned on them that excessive mGluR activation in fragile X could potentially give rise to multiple symptoms of the disease. In fragile X patients where FMRP was gone, they reasoned, inhibiting mGluR5 might treat the condition.
With no reputation in the fragile X field, Bear nevertheless dared to present the idea at a meeting of experts.
“I come up with this outlandish idea and I just absolutely remember there was a stunned silence at the end of the talk,” Bear said. “You could just see everybody rubbing their chin, as if thinking ‘this idea is so out there it’s got to be wrong and yet we can’t immediately think of a reason why it’s wrong’.”
“You could just see everybody rubbing their chin, as if thinking ‘this idea is so out there it’s got to be wrong and yet we can’t immediately think of a reason why it’s wrong’.”
That kind of doubt and silence was all he would encounter in those days trying to fund his nascent hypothesis through traditional means. Instead he was able to persist because of open-ended funding he had earned from the Howard Hughes Medical Institute and because FRAXA, a private foundation that funds fragile X research, had agreed to fund a fellowship for Huber. FRAXA continues to fund Bear’s research, which has now yielded several drugs worthy of testing in stage III clinical trials..
Trying ideas in new ways
Much as Bear was not known in Fragile X circles, Myriam Heiman has not yet published much about Parkinson’s disease or schizophrenia. She has, however, helped to develop TRAP, an innovative method for studying the unique patterns of gene expression among different cells in the brain. She has also achieved the first ever genome-wide screen in a mammalian brain to discover which genes help cells fight back against the toxic protein that causes Huntington’s disease. That involves disabling each gene in the entire mouse genome, one gene per neuron, in an area of the brain highly affected in disease to see which genes’ absences make neurons more vulnerable. Recognizing that these methods might help in the study of schizophrenia and Parkinson’s disease respectively, she has embarked on studies to try.
The schizophrenia research is supported by Eduardo Elejalde-Arena, founder of the Natalia Mental Health Foundation named for his daughter who had schizophrenia. With Elejalde-Arena’s support and further funding from JPB, Heiman has been using TRAP to determine which cells antipsychotic drugs such as clozapine affect to help patients, and exactly how those neurons respond to it. Learning this precise mechanism could help in designing new drugs that are more effective and have fewer side effects.
And after discovering which genes are necessary to endow vulnerable cells with defenses against Huntington’s disease, Heiman is embarking on a new study to determine whether her technique could do the same for the study of Parkinson’s disease. That untried idea is proceeding with funding from the Mathers Foundation.
It’s much too soon to say whether Heiman will find anything that ends up showing promise in clinical trials, like Bear and Tsai’s discoveries have done, but that’s exactly the point. These are new ideas. There is a risk they won’t work. But with funders willing to take that risk alongside Picower Institute researchers, innovative science has launched and soared many times before.