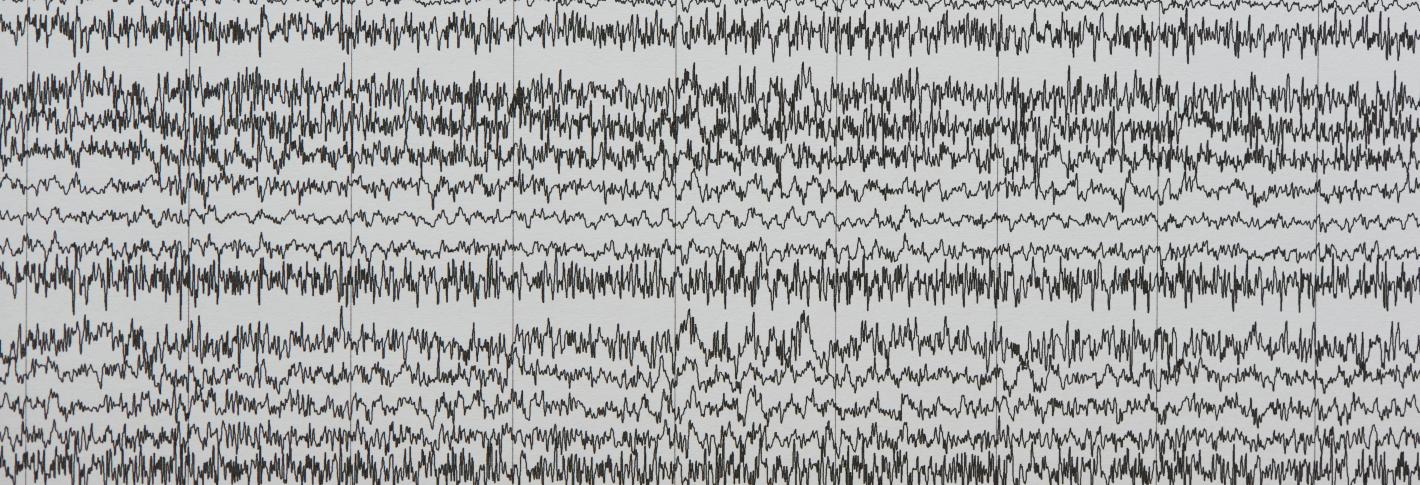
By making and analyzing measurements of thousands of neurons and surrounding electric fields in three cortical regions in animals, the team’s new study in the Journal of Cognitive Neuroscience provides a unifying view of how brain waves, which are oscillating patterns of the activity of brain cells, may control the flow of information throughout the cortex.
“When you look at prior studies you see examples of what we found in many regions, but they are all found in different ways in different experiments,” said Earl Miller, Picower Professor of Neuroscience in The Picower Institute for Learning and Memory and senior author of the study. “We wanted to obtain an overarching picture so that’s what we did. We addressed the question of what does this look like all over the cortex.”
Added co-first author Mikael Lundqvist of Stockholm University and MIT: “Many, many studies have looked at how synchronized the phases of a particular frequency are between cortical regions. It has become a field by itself, because synchrony will impact the communication between regions. But arguably even more important would be if regions communicate at different frequencies altogether. Here we find such a systematic shift in preferred frequencies across regions. It may have been suspected by piecing together earlier studies, but as far as I know hasn’t been shown directly before. It is a simple but potentially very fundamental observation.”
The paper’s other first author is Picower Institute postdoc Andre Bastos.
To make their observations the team gave animals the task of correctly distinguishing an image they had just seen – a simple feat of visual working memory. As the animals played the game, the scientists measured the individual spiking activity of hundreds of neurons in each animal in three regions at the bottom, middle and top of the task’s cortical hierarchy – the visual cortex, the parietal cortex and the prefrontal cortex. They simultaneously tracked the waves produced by this activity.
In each region they found that when an image was either being encoded (when it was first presented) or recalled (when working memory was tested), the power of theta and gamma frequency bands of brain waves would increase in bursts and power in alpha and beta bands would decrease. When the information had to be held in mind, for instance in the period between first sight and the test, theta and gamma power went down and alpha and beta power went up in bursts. This functional “push/pull” sequence between these frequency bands has been shown in several individual regions, including the motor cortex, Miller said, but not often simultaneously across multiple regions in the course of the same task.
The researchers also observed that the bursts of theta and gamma power were closely associated with neural spikes that encoded information about the images. Alpha and beta power bursts, meanwhile, were anti-correlated with that same spiking activity.
While this rule applied across all three regions, a key difference was that each region employed a distinct peak within each frequency band. While the visual cortex beta band, for instance, peaked at 11 Hz, parietal beta peaked at 15 Hz and prefrontal beta peaked at 19 Hz. Meanwhile visual cortex gamma occurred at 65 Hz, parietal gamma topped at 72 Hz and prefrontal gamma at 80 Hz.
“As you move from the back of the brain to the front, all the frequencies get a little higher,” Miller said.
While both main trends in the study – the inverse relationships between frequency bands and the systematic rise in peak frequencies within each band – were both consistently observed and statistically significant, they only show associations with function, not causality. But the researchers said they are consistent with a model in which alpha and beta alternately inhibit, or release, gamma to control the encoding of information – a form of top-down control of sensory activity.
Meanwhile, they hypothesize that the systematic increase in peak frequencies up the hierarchy could serve multiple functions. For instance, if waves in each frequency band carry information, then it higher regions would sample at a faster frequency to provide more fine-grained sampling of the raw input coming from lower regions. Moreover, faster frequencies are more effective at entraining those same frequencies in other regions, giving higher regions an effective way of controlling activity in lower ones.
“The increased frequency in the oscillatory rhythms may help sculpt information flow in the cortex,” the authors wrote.
The study was supported by the National Institutes of Health, the Office of Naval Research, The JPB Foundation, the Swedish Research Council, and the Brain & Behavior Research Foundation.